Structure and function of satellite positioning systems (GNSS)
Satellite positioning systems are commonly referred to as GNSS. The abbreviation stands for Global Navigation Satellite System and describes a complex of satellites and ground stations with which the positions and directions of movement of stationary or mobile receiving units (“GPS receivers”, “GNSS sensors”) can be determined based on signal transit time measurements.
The best-known GNSS is the American GPS (Global Positioning System). In addition to GPS, various other GNSS such as GLONASS, GALILEO and COMPASS are operated or built.
Space component
Satellites play a central role in GNSS. They essentially consist of a power supply, an atomic clock and transmitter and receiver units for communication with the ground segment and the transmission of location signals. GNSS satellites regularly transmit signals in the frequency range 1.2 to 1.6 GHz that contain a timestamp (PRN code) and a unique identifier of the satellite (SVN – space vehicle number).
So-called ephemerides are sent out at longer intervals. These contain information about the satellite’s orbit data and enable a GNSS sensor to determine the satellite’s current position in space.
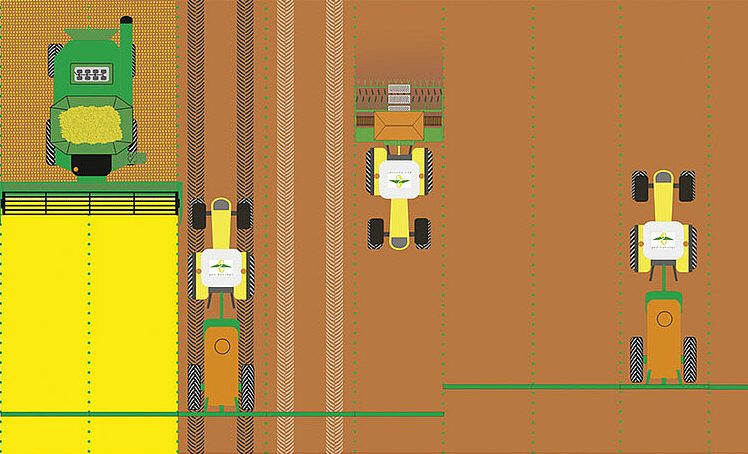
Soil component
The ground component of a GNSS consists of various control stations that monitor the trajectory of the satellites and correct them if necessary. The ground control stations also monitor the correct functioning (integrity) of all satellites belonging to the GNSS and the entire system. In the event of an error, individual satellites are marked as non-functional (unhealthy) and are then not taken into account by GNSS sensors when calculating the position.
The control stations determine deviations from the expected trajectory of satellites and their atomic clocks and regularly summarize the current orbit data and clock errors in the form of so-called ephemerides (or almanacs). The control stations make the ephemeris available to the receiving devices via the satellites.
Principle of runtime measurement The position determination with GNSS is similar to the position determination with sex tanks. With the help of sextants, the angles between stars are determined. From three angular measurements, the position can be calculated (triangulation). At position measurement with GNSS, the positions of three distance measurements are derived (trilateration).
The satellites transfer a timestamp of the integrated atom clock next to their unique identifier. After the signal has arrived at the receiver, it determines how long the signal for its journey has needed from the satellite to the antenna (runtime). Based on the assumption that the signal is spread with light speed, the distance between the satellite and the antenna can be calculated from the runtime and the speed of light (pseudo Range). The position of the satellites in space is known to the GNSS receiver from the ephemerides and from three positions and three distances can the position of the antenna be clearly determined – comparable to a ball which remains at three tight threads from the ceiling hanging in a position.
Construction and operation of GNSS sensors
Antenna and receiving unit can be integrated in a housing. One speaks then from a Smartantenne. The goodness of GNSS antennas differ significantly due to their design and quality. The accuracy of a GNSS sensor is therefore not only influenced by the recipient, but also decisively by the antenna.
A occasional, the receiving units are integrated into the operating terminals of steering systems. The antenna is then removed on the roof. This design offers the advantage that steering systems can be converted faster from a vehicle to another. GNSS receivers used in vehicles are usually supplied with power over the 12V electrical system. When used as a worn mapping system, the supply is carried out via an external 12 V battery.
The computers have internal batteries, which also supply the GNSS sensor. The antenna is usually also integrated with these devices. The accuracy of GNSS sensors integrated in hand writers is usually limited by the quality of the antennas. 3.2 Features of GNSS sensors
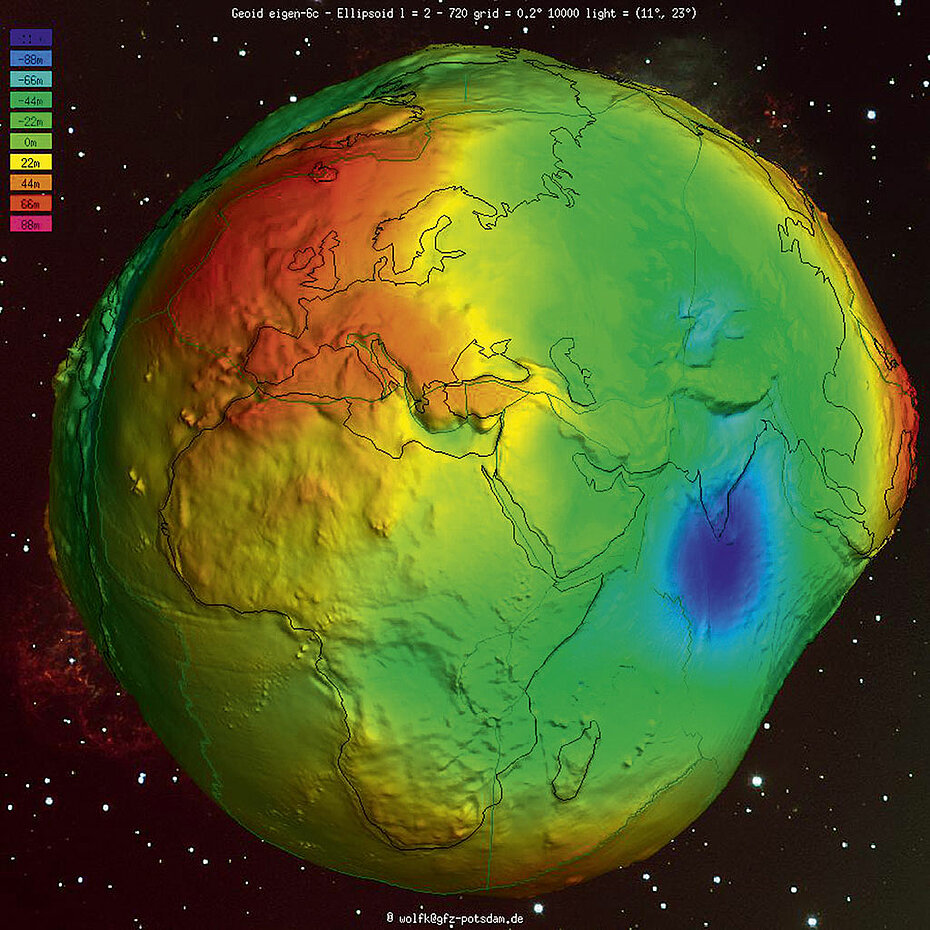
Measured values of a GNSS sensor
GNSS sensors determine the three-dimensional position of their antenna by measuring the transit time of satellite signals.
The height measurements refer to the WGS 84 ellipsoid, a mathematical model that efficiently, but not exactly, describes the shape of the earth. When exaggerated, the earth looks like a potato due to the changing structure of the earth’s crust.
The actual shape of the Earth is called a geoid. Most GNSS sensors output the local deviation between the ellipsoid and geoid in addition to the ellipsoid height. This value is called geoid separation and allows the height above sea level (NN) or mean sea level (MSL) to be calculated.
Digital terrain models (DGM, DTM) can be created from the height measurements using suitable software, such as most yield mapping programs. These terrain models can in turn serve as a basis for the assessment of partial areas (water balance, nutrient input, radiation benefit).
Measured values of a GNSS sensor
In addition to position and altitude, GNSS sensors also determine speed very precisely. The speed is not calculated from the transit times of the signals, but from the frequency shift (Doppler effect). Speed measurement with GNSS is significantly more accurate than measurements from radar and wheel sensors, especially at low speeds. The measured values are therefore very suitable for controlling speed-dependent processes (quantity control of fertilizer spreaders, crop protection sprayers, sowing and planting machines).
3.2.2 Accuracy
The accuracy of GNSS sensors depends on various factors. In addition to the quality of the antenna, signal filtering, and the signal processing algorithms, the number of available satellites, the constellation of the satellites and the extent of the atmospheric disorders in the ionosphere are decisive. In addition, errors in the determination of the satellite edges and watch errors affect the accuracy of GNSS. Since the influence of the atmosphere and the satellite constellation are constantly changing, no reliable statements for the accuracy of a GNSS sensor can be derived from a measurement performed over a short period of time. Long-term and repeated test measurements result in statistical key figures that describe the accuracy of a GNSS sensor. The accuracy is usually given as RMS (65-68%) or 2 RMS (95%).
Absolute accuracy (deviation from the true position) is not relevant for all applications. For many agricultural applications, the relative accuracy, based on the last position or positions, is the crucial measure. If areas are measured solely with the aim of determining their size, the absolute positioning accuracy of the GNSS sensor used is irrelevant. If all positions recorded while traversing the area have a consistent offset in the same direction, the size of the area is accurate, even though the position of the outline is offset from the real location of the field boundary. For classic parallel guidance (e.g. preemergence herbicide application), it is not the absolute position of the lanes that is crucial, but rather their distance from one another. As long as this distance corresponds to the working width of the field sprayer, overlaps and imperfections are avoided, regardless of the absolute accuracy of the position measurement. Therefore, when specifying the accuracy, a distinction is made between absolute accuracy (deviation from the true position) and relative accuracy (deviation from the last measured positions).
GNSS sensors usually output the position data and other measured values determined by the sensors such as time, date, speed and direction of travel via a serial interface (RS 232). The data is transmitted as ASCII plain text in NMEA 0183 format. The NMEA messages are read by computing units and used for measuring points, spatially recording sensor readings or controlling (e.g. steering, site-specific application).
Selected GNSS sensors are able to output their measured values on a CAN bus. The data output then usually follows the NMEA 2000 standard or the ISO 11783 standard (parts 3 and 5). For transmission in commercial vehicle construction, isolated measured values are defined in the J1939 standard. The advantage of transmitting GNSS measured values via the CAN bus is the possibility of supplying different subsystems (steering, section switching, quantity control) with data using a single physical interface